Funded by $1.5M DOE grant, Lehigh’s Plasma Control Group embarks on new project at the National Spherical Torus Experiment Upgrade (NSTX-U) that will contribute to eventual design of energy-producing tokamak reactors
How will the future of fusion-powered nuclear energy take shape?
It could boil down to a question of apples versus donuts.
For scientists and engineers trailblazing toward the still-long-off goal of a sustainable, safe, and economically feasible fusion reactor, the choice represents two different design approaches to tokamaks, the giant devices used to study the behavior of fusion plasmas.
“Conventional tokamaks, like the DIII-D National Fusion Facility in San Diego, have this toroidal, or donut, shape,” says Eugenio Schuster, a professor of mechanical engineering and mechanics in Lehigh University’s P.C. Rossin College of Engineering and Applied Science.
Spherical tokamaks, such as the National Spherical Torus Experiment Upgrade (NSTX-U) at Princeton Plasma Physics Laboratory (PPPL) in New Jersey, “while still technically a donut shape, have a very small hole in the middle,” he explains, “so they look more like a sphere.” (Visualize a cored apple.)
Schuster leads Lehigh’s Plasma Control Group, which was recently awarded more than $1.5 million in funding from the U.S. Department of Energy’s Office of Fusion Science to conduct research at NSTX-U. The work will help physicists answer key questions about the spherical tokamak concept and contribute to the eventual design of a compact, efficient fusion reactor.

Tokamaks confine a plasma—the same superheated electrically charged gas where nuclear fusion takes place in the sun and the stars—within magnetic fields. This “invisible bottle” prevents the plasma (at around 100 million degrees Celsius) from touching the inner wall of the tokamak. In present tokamak “devices” like DIII-D and NSTX-U, researchers are focused on studying the physics of the plasma while avoiding a significant amount of nuclear reactions that would generate undesirable levels of radioactivity. Understanding the dynamics of this plasma will enable the development of tokamak “reactors,” where energy generation will be the goal.
NSTX-U is in the process of coming back online following a shutdown caused by a malfunctioning magnetic coil in 2016, when it was resuming operations after a four-year upgrade. Schuster says he is confident in the re-engineered device and is looking forward to using its upgraded capabilities to explore the possibilities of the spherical tokamak geometry.
“For Lehigh to be part of this relaunch, it is an honor for us,” he says. “And we're very excited about what this machine will be able to do.”
“Our project is a mix of plasma physics work and control science work,” he explains. “One of the priorities for NSTX-U is to get what we call advanced plasmas, which have greater stability and can work longer in a steady state, meaning without the help of inductive means of current. And they have high performance, mainly measured in terms of pressure. The higher the pressure, the better, because the more efficient the nuclear fusion reaction will be.”
Schuster and his multidisciplinary team will develop models to better understand the dynamics of these types of plasmas and write physics-based codes to predict their behavior. The models will lead to the design of control strategies to effectively regulate the plasma in closed loop.
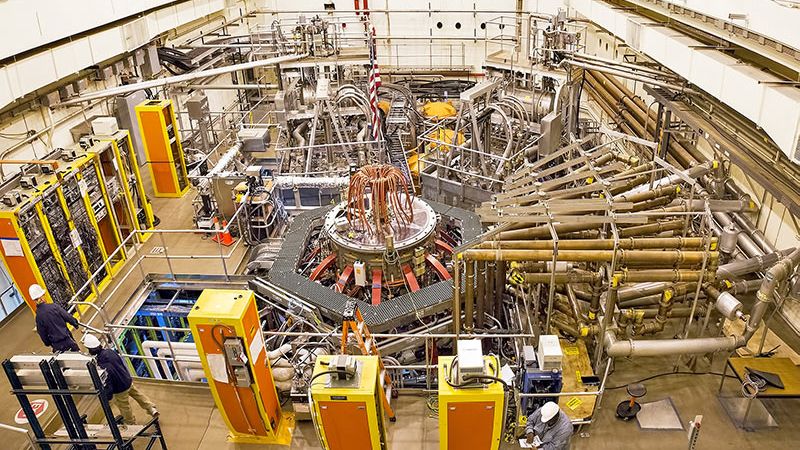
The collaboration will include Tariq Rafiq (principal research scientist in the mechanical engineering and mechanics department), grad students, and a postdoctoral researcher, along with one of Schuster’s former PhD students, Mark Daniel Boyer, a staff research scientist at PPPL.
“I love to have my own students become first postdocs and later staff scientists at these facilities,” he says. “Being part of these types of projects puts them in a privileged position, because when they graduate, they have already experienced running experiments on these big devices at these first-class research facilities. It’s not common for PhD students to have these opportunities, which I find very effective and educational.”
In addition to his efforts at DIII-D and NSTX-U, Schuster conducts research on tokamaks around the world, including KSTAR in South Korea and EAST in China, with students often working alongside. The coronavirus pandemic has added additional challenges and put a halt to travel, though he says experiments have and will continue to be run from afar.
“Even before the pandemic, when we had the possibility of running experiments on the machines for just a few hours, it wasn’t worth traveling, so we would do them remotely by setting up the control system that tells how the machine is run in each discharge. The virus makes everything more difficult, but we keep moving forward.”
For Schuster, looking to the future is part and parcel of his research. As a Scientist Fellow in Plasma Control for ITER (the world’s first nuclear reactor based on fusion, currently under construction in France), he is part of the effort to get the facility operational by its 2025 target. From there, it will likely take another 10 to 15 years to produce energy from fusion at the reactor’s intended target of 10 times the input power required for operation.
His experiments at NSTX-U take an even longer view.
“At this moment, we're looking into the potential benefits of a spherical design. But if we demonstrate that there are indeed benefits, the next reactor will be different from ITER. Instead of being in a classical toroidal configuration, it will be more in this kind of more compact spherical configuration that we intend to explore at PPPL.”
—Katie Kackenmeister is the assistant director of communications for the P.C. Rossin College of Engineering and Applied Science