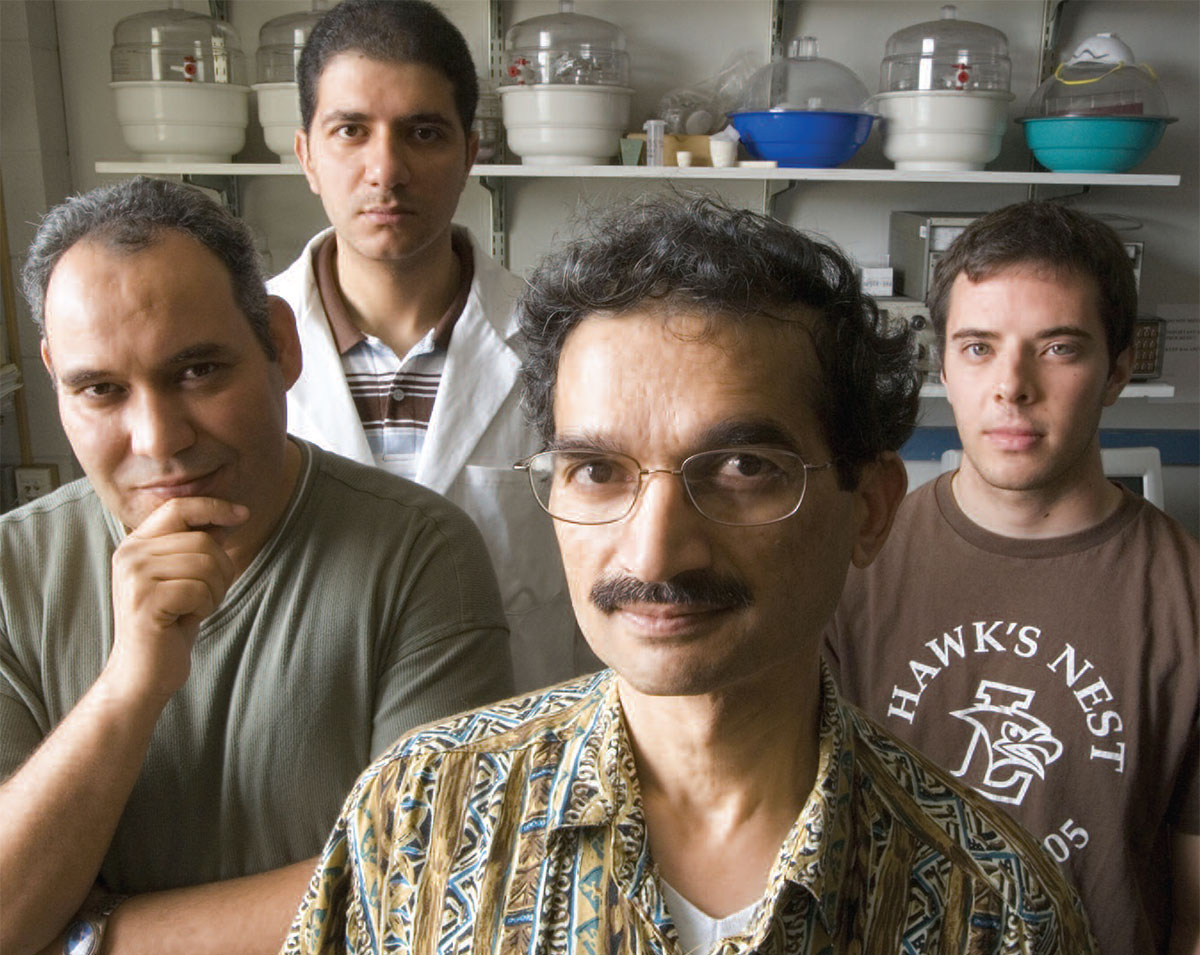
Glass, it seems at first glance, is almost endlessly versatile. We use glass to repair our eyesight, to adorn skyscrapers and to create artistic treasures. We employ it to reflect, refract and transmit light, and to exchange unthinkable quantities of information in slices of seconds. But all this is just the surface, says Himanshu Jain. Someday, if we can learn to tailor its chemical and mechanical properties, glass may be used to mimic and even restore the miracles of nature.
Jain, director of NSF’s International Materials Institute (IMI) for New Functionality in Glasses at Lehigh University, has spent three decades exploring the myriad uses of glass.
He accepted a new challenge during the 2004 U.S.-Africa Materials Workshop in Cairo, when he took a break to visit the University of Alexandria on Egypt’s Mediterranean coast.
Mona Marei, head of the tissue-engineering lab at the university’s Faculty of Dentistry, had asked Jain for help with a growing medical problem – the deterioration of people’s teeth and jawbones. Osteoporosis, she told Jain, was causing the bone around people’s teeth to weaken, making it difficult for doctors to replace loosening or diseased teeth with prosthetics or implants and, in severe cases, causing fractures that required removal of bone and teeth.
Marei was experimenting with biocompatible glass bone transplants to replace damaged teeth and bone, but was not satisfied with her results.
Biocompatible materials can be placed inside the body without triggering a systemic reaction or an infection. But Jain suspected something more than biocompatibility was required of the glasses that Marei was testing.
The ideal treatment for diseased or damaged bone is to coax the body’s natural bone tissue to regrow. Doctors have succeeded in taking a bone graft from one part of a person’s body and using it as a “scaffold” to stimulate bone tissue elsewhere to regrow. But although biocompatible glasses have been used as bone transplants, no one has yet succeeded in using glass as a bone scaffold.
Until now.
Today, working with researchers in four countries on three continents, Jain and his collaborators have modified a biocompatible glass belonging to the calcium-phosphatesilicate family into a scaffold that promises to stimulate bone regeneration. They have successfully tested the glass in in vitro experiments at Lehigh’s IMI. Marei and her group are preparing to run in vivo tests at the University of Alexandria.
An overseas assist with dual porosity
In seeking an effective scaffold for bone regeneration, Jain and Marei sought to develop a glass that would promote transport of nutrients and blood while allowing new cells to “in-grow” and adhere to the scaffold.
Like the spongy interior of bone, the new material would need interconnected pores to facilitate the production of red blood cells and the flow of blood to other areas of the bone.
A glass scaffold might emulate the mechanical properties of bone, Jain believed, if it could be made porous at both the nano and macro scales.
Nanopores measuring several nanometers in diameter (1 nm equals one one-billionth of a meter) would allow cell adhesion and crystallization of bone’s structural components.
Macropores measuring in the tens of microns or larger, roughly 10,000 times the size of nanopores, would allow bone cells to grow inside the scaffold and to vascularize, or form new blood vessels and tissue.
To achieve “dual porosity” in a biocompatible glass, Jain turned to two researchers he knew from the Instituto Superior Técnico in Lisbon, Portugal – Rui Almeida, professor of materials science and engineering, and Ana Marques, a research scientist who has studied pore formation while preparing wave guides for optical amplifiers.
During a recent stay at Lehigh’s IMI, Marques used common lab equipment, including a ventilated hood and a hot plate, to develop glass with dual porosity. She employed standard experimental techniques – nitrogen adsorption and porosimetry to measure material surface areas and poresize distributions and high-resolution scanning electron microscopy (SEM) to detect pores.
Marques also employed the sol-gel process, a wet chemistry technique that uses relatively low temperatures to prepare glass. After mixing a solution, she allowed it to gelate and then heat-treated the gel until it formed a glass.
The sol-gel process inherently promotes nanoporosity, says Marques. To achieve interconnected macroporosity, Marques added a polymer to the sol-gel solution, causing a phase separation to occur parallel to the sol-to-gel transition.
The phase separation enabled Marques to overcome a simple law of thermodynamics, says Jain.
“Thermodynamically, the coexistence of nanopores and macropores is unstable in that the larger pores should absorb the smaller pores,” he says. “But with Ana’s help, we have developed a material that defeats that expectation.”
After three months at Lehigh’s IMI, Marques was able to produce glass with nanopores measuring 5 to 20 nm in diameter and macropores measuring more than 100 microns across.
“The aim of our project was to create nano- and macroporosity in a bioactive material while achieving mechanical properties that match those of bone,” says Marques. “We believe our material will stimulate bone regeneration because cells will proliferate inside the scaffolding material and form tissues, thus facilitating the delivery of nutrients to regenerating bone tissue.”
“When you attach the glass to the damaged bone,” says Mohamed Ammar, a dentist and research scientist at the University of Alexandria’s tissue-engineering lab, “a layer forms on the surface of the glass that has the same chemical composition as the natural bone. The bone cells come to this layer and attach to it, in effect, forming a bone matrix around the glass.”
As the treated bone regenerates, says Marques, the scaffold, being biodegradable, is absorbed by the body.
“This is the next generation of biomedical applications,” says Marques. “It will be helpful for all bones, ranging from teeth to arms and legs. It can be used for breaks, tumors and other defects.”
“This kind of material,” says Jain, “might have other applications, such as controlled drug delivery, which could be achieved when a drug is released at differing rates because of the varying porosity.”
The scaffolding material, says Ammar, who is now helping Marei supervise in vivo tests in Egypt, offers new hope to his patients, many of whom are women suffering from osteoporosis.
“We’ve been giving our patients medicines and prescribing exercises to strengthen their bone tissue and slow down the disease,” says Ammar, who recently concluded four months of research at Lehigh’s IMI. “This gives us something new. It is personally very exciting.”
Two techniques in a friendly duel
While the Lehigh IMI team is applying for a patent on its sol-gel technique, it is also testing a second technique for producing glass with dual porosity.
The melt-quench technique, also called the melt-and-pour technique, is the traditional process for making glass, says Hassan Moawad, who leads a Lehigh group that is modifying the technique to achieve dually porous glass (see story, p. 13). Moawad is also a member of the materials science faculty at the University of Alexandria.
“This is a friendly competition between two groups,” says Jain, “that will enable us to compare and contrast the efficiency of the two techniques.”
The researchers in Moawad’s group use standard glassmaking techniques, weighing and mixing the oxide powders, melting them at 1500 degrees Celsius, then grinding and polishing the glass and cutting it into small discs. The heat treatment stimulates crystallization, which strengthens the glass. Researchers also use a chemical treatment to etch the glass and introduce desired porosity, x-ray diffraction to measure the degree of crystallization and hardness, and optical microscopy and SEM to characterize the glass.
The melt-quench technique produces a stronger, more solid glass than does the sol-gel process, says Moawad. Glass made with the melt-quench technique can also be more readily molded.
But glass made with the sol-gel technique appears to be more biocompatible than melt-quench glass, says Moawad.
Moawad’s team has succeeded in producing glass that is porous at the nano- and macro-scales. They have used SEM to verify pore diameter and surface area, along with BET (Brunauer-Emmett-Teller), a technique that measures nanopore dimensions, and mercury porosimetry, which measures slightly larger pores.
A key to the team’s success, says Moawad, was discovering the correct proportion of oxides in the glass powder mixture. Moawad worked one year to develop the most effective mixture of silicon, calcium, phosphorous and boron oxides.
The next step for the researchers, says Jain, is to investigate the mechanical properties and bioactivity of the new materials, as well as the responses of cells that interact with the material.
The Lehigh-Lisbon-Alexandria research team is working on these challenges with researchers from Princeton University through the U.S.-Africa Materials Institute, which is headquartered at Princeton and directed by Wole Soboyejo, a materials engineer at Princeton.
A graduate student from Senegal will come to Lehigh later in 2006 to work on the project, says Jain.