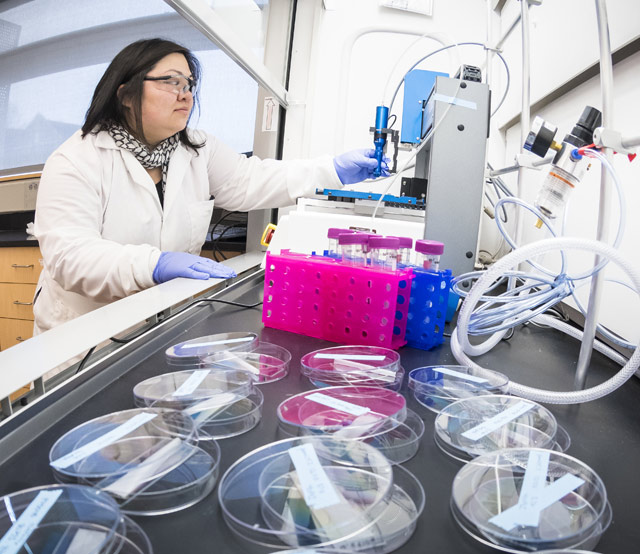
Yaling Liu recalls the moment he transformed from a mechanical engineer into a cancer warrior.
“I was in Washington, DC, in August of 2011, preparing to participate in a panel discussion,” says the associate professor and member of Lehigh’s Bioengineering program. “I had a free morning before my meeting, and decided I’d spend it in a National Institutes of Health conference that happened to be taking place at a nearby hotel.”
The conference he’d stumbled upon was focused on health technology in the developing world. Yaling, seeking nothing more than to satisfy personal curiosity, had taken a seat in a session highlighting a low-cost, mobile device for cancer screening.
“The talk was being led by public health experts and clinicians,” he says. “With my background in fluid mechanics and engineering, I couldn’t help but realize there were fundamental techniques that could be employed to improve upon an already-impressive design.”
Yaling spent his first few moments as a cancer researcher in the back of a crowded conference room, scribbling out computations on the back of a hotel envelope: an inauspicious beginning for what has developed into a promising and amazingly inexpensive technique for isolating and detecting tumor cells in the bloodstream.
Engineering affordable, accessible health
Targeted nanotherapies that pinpoint and destroy pathogens while leaving healthy tissue unscathed. Metal foams and synthetic lattices that reinforce vulnerable body parts and promote healing. Wireless diagnostic tools implanted in the body that alert wearers to potential problems.
Wojciech Misiolek says that such ‘miracles’―as well as other innovative recent advances in health technology―are brought about through the creative incorporation of expertise from across a spectrum of fields related to science, technology, engineering, math, and design. “At Lehigh, multidisciplinary is in our proverbial DNA,” says Misiolek, chair of materials science and engineering and director of the Loewy Institute at Lehigh. “We actively seek out and foster expertise at the intersection of disciplines. So engineers here are ‘genetically predisposed’ to contributing to this emerging field, and our tradition of leadership in materials and mechanics serves as a powerful foundation for innovation.”
“We have a long history of interdisciplinary work here at Lehigh,” continues Gary Harlow,professor and chair of mechanical engineering and mechanics. “Collaboration that exploits multiple technologies is liable to push a researcher out of a typical comfort zone, so we attract people who are OK with that.”
“The development of technology for medical usage brings added layers of complexity to traditional areas such as electrical, materials and mechanical engineering,” explains Anand Jagota, a professor of chemical and biomolecular engineering and director of Lehigh’s Bioengineering program. “For example, if you are going to grow a tissue or design an implant for the human body, you must understand the tissues that will absorb it. Your implant needs to have the mechanical or electrical capability to safely complete its task, and have the correct size, shape, and distribution of pores to accommodate the movement of nutrients and cells. Plus, if you want the implant to degrade at a specific rate, you must also understand how its location and usage in the body impacts its effectiveness and the healing of the tissues surrounding it.
“A solution that handles all of the necessary considerations couldn’t possibly derive from a single perspective.”
“The most interesting science in our era will be done at the borders between disciplines and by exploring the features of these largely unmapped regions,” says John Coulter, professor and associate dean for research and operations. “In this biomedical materials field, five years from now the scenery will be entirely different than it is today, which is entirely different than it was five years ago. So, we develop people who will be able to figure out how to make contributions in an ever-evolving space.”
“It’s a misconception that an engineering degree ‘locks you in’ on a specific career path,” he says. “Once you graduate or earn that Ph.D., the specific field tends to get less important. People will want to know: What are you doing now? How are you leveraging your skills to make a difference?
“The idea of coming to Lehigh and staying in a silo for an entire career isn’t an option,” he continues. “Here, it’s a requirement to blend groups across disciplines, and that’s especially evident in our bioengineering and health initiatives. We rely on that approach in all we do, and at the end of the day that mentality creates a wonderful benefit for our faculty and students.”
A perfect collaboration
Spend some time with Lesley Chow and Hannah Dailey ‘02 ‘06G ‘09 Ph.D., and it will soon be abundantly clear that they make a great team. Chow, an assistant professor of materials science and engineering affiliated with Lehigh’s Bioengineering program, and Dailey, an assistant professor of mechanical engineering, are both new members of Lehigh’s faculty. They met at a new-hire orientation session―serendipity borne of Human Resource procedure―and now work together, and with a local hospital network, designing bioactive tissue scaffolds that can biodegrade as new tissue regenerates.
“Lesley and I are the perfect example of a Lehigh collaboration,” says Dailey. “What we are working on is really exciting because it touches on everything from the design of materials to execution, physiological loading, mechanical design, and surgical implementation. Lehigh is really committed to investing in these areas and bringing together skill sets.”
Their tunable tissue engineering project leverages Dailey’s experience with orthopedic devices and Chow’s knowledge of biomaterials. In their lab, they are building customizable lattices with bioactive surfaces, using a computerized robot that was customized into a specialized 3D printer. When the lattices are inserted in the body, the polymer scaffolding provides structural integrity, while bioactive functional groups attached on the surface attract the types of cells needed to promote the desired healing activity.
The lattices can be custom built for size and for a desired rate of biodegradation, depending the polymer used. Varying biochemistry can also be presented on the lattices, depending on the physiological process needed.
“The benefit of our technique is that it’s universal,” says Chow. “We can look for segments of proteins to find the biological cues we need, and put them on the polymers before we print the lattice. The implant then presents these cues to the body in a biologically appealing way. And if we find out a particular molecule isn’t working, we can change the chemical cue to foster the result we want.”
One way of imagining the project is like 3D printing, with a twist. “Right now, 3D printing is a buzzword,” Chow says. “We are riding that wave, but taking a slightly different approach by looking at the research from all angles. The processing affects materials, and vice versa, and this has an impact upon the cells themselves. So, we are getting down to the nitty-gritty and finding out what that cell will feel when it encounters our scaffold.”
At present, Chow and Dailey are working toward clinical applications that include tendon, ligament and cartilage repair, but their ambitions reach higher.
“The platform we’re developing can build polymeric structures that induce regeneration of tissue, and we will be able to tweak its parameters and apply it to lots of different clinical therapeutic areas,” says Dailey. “Medical treatment for orthopedic injuries and degenerative joint disease can be incredibly difficult for patients, and places a tremendously expensive burden upon the U.S. healthcare system. We think our technology can make a real difference.”
The team has received a Collaborative Research Opportunity grant from Lehigh and are partnering with the Department of Orthopaedic Surgery at St. Luke’s University Health Network in Bethlehem.
“This project allows us to share our expertise, bring out the best in our work and look to the future,” says Chow. “It’s one of the benefits of being here at Lehigh.”
Unlocking mysteries on a breathtaking scale
Faculty researchers Sabrina Jedlicka and Slava V. Rotkin began collaborating at the instigation of a student of Rotkin’s seeking greater academic exposure to biophysics and materials engineering.
Rotkin, a professor of physics in the College of Arts and Sciences with a joint appointment in materials science, turned to Jedlicka, an associate professor of materials science who serves as a core faculty member in the University’s Bioengineering program, for help.
“I think we had talked to each other three times before that,” notes Jedlicka, laughing, whose work studies the fundamental interactions between cells and substrates, with a specific focus in neurobiology.
The student’s interest bloomed into an ongoing faculty-led exploration into the dynamics of nanotubes wrapped in DNA, and the fundamental physics and behavior of this hybrid material within cells.
“When nanotubes wrapped in DNA are introduced into a stem cell, the differentiation rate for that cell increases tenfold,” Jedlicka reports. “The rate of differentiation from stem cells to neurons was remarkable, and encouraged us to further pursue therapeutic possibilities.”
“The brain is the last frontier,” she continues. “I’ve worked a lot with neuronal differentiation, taking a stem cell and changing it into something more mature. There are so many people suffering from brain diseases, and we need to think about alternative therapies that will improve the quality of life for people suffering from these ailments.”
Her project with Rotkin combines nanoscale materials science and bioengineering in ways that have broad possibilities for biomedical therapies.
“We are not using active DNA,” says Jedlicka. “It is biologically inert and doesn’t encode for anything; we believe the outcome has more to do with DNA keeping the nanotubes from clustering and thus clogging inside the cells.”
Interestingly, the project is one of the first to examine nanotubes within cells in pharmacologically relevant doses. Much of the prior research has used massive doses that are toxic to cells―for the simple reason that it made the nanotubes easier to find. “The approach is understandable from a materials research perspective,” says Jedlicka, “but the medical requirement to ‘first do no harm’ is paramount. So we had to find a different way.”
A cell is about 10,000 times larger than a nanotube, and thus one of the challenges to their research is getting a look at the interaction. Rotkin has vast experience in nanocharacterization, a field devoted to finding ways to, literally, make the invisible visible. “This is a big problem,” says Rotkin. “At this scale, your tools are bigger that the thing you are looking at. You have to be creative and find ways to beat the limits of nature―the wavelengths of light are bigger than what we are seeking!”
The technique Rotkin employs, Raman microscopy, uses hyperspectroscopic measurements to build a kind of multidimensional map of areas in the cell. It is a slow, tedious process, demanding multiple passes to generate each image point by point. But it has been successful, and they believe they have captured clear images of single nanotubes inside of cells.
Rotkin and Jedlicka have received seed funding from Lehigh to support this research, which has proved critical to laying the groundwork for further study on nanomaterial-cell interactions.
“That is very important,” he says, “and for cross-disciplinary science the importance is squared or cubed. There is interesting physics and biology here, precisely because we are pushing the boundaries in this way. We’ve now been able to build some preliminary, supportive evidence that tells a much stronger story to potential funding and research partners.”
Cancer detection for less than latte
Yaling Liu, a specialist in nanomechanics who serves as associate professor of mechanical engineering, has developed technology to detect cancer long before a tumor would be noticed by MRI or other existing techniques. It could also be used to monitor the effectiveness of cancer treatment.
The material cost for the device is under a dollar, and Liu anticipates a complete device in the $5 range. Its low cost raises the potential that it could be used for home testing or deployed in the developing world.
Liu’s foray into the materials for medicine occurred when he attended a conference on affordable medical devices several years ago. In simple terms, Liu applied his expertise in computational fluid mechanics and nanofabrication to come up with a chip that can detect certain tumor cells in the blood. The ‘lab-on-a-chip’ his team has developed works by a running a fluid sample across a specially formulated surface where pathogens, such as circulating tumor cells, or CTCs, are trapped and identified. The chips are made with transparent polymers, and the polymer surface is structured so that it can be populated with antibodies able to capture the tumor cells.
“The unique thing is the design of the capture bed on the surface of the chip,” says Liu. “The capture bed is in the substrate, and has both microscale and nanoscale features that are engineered to enhance capture efficiency. Cells have protruding patterns, so we have tried to create patterns on the capture pad that are complementary to the patterns on CTCs. The herringbone surface we employ generates a passive vortex that mixes the cells, and increases the chance that CTCs will collide with the capture pad. We're also adding some other nanoscale features like pillars or nanospheres that are complementary to the surface components of those tumor cells.”
Much of Liu’s current research interests involve mimicking animal or human tissue function to develop new testing and diagnostic tools. In that vein, Liu is creating a technique to speed and enhance pharmacological testing with a device that mimics blood vessels and blood flow.
“There is established, detailed data on ranges of blood flow,” he says. “We culture different types of tissues together to recreate blood vessels, and then we can inject a very low quantity of a drug and track the response. We'll also be able to culture human cells in 3D, rather than in a two-dimensional culture dish.”
Current animal or human drug testing does so one condition at a time; a device as envisioned by Liu would allow for high-speed concurrent testing for hundreds of different conditions.
“My original training is mostly in mechanical engineering,” says Liu, “and personally I feel like the medical field is an often-overlooked application area of my home discipline. The tools I generally use hail from the world of mechanical engineering and mechanics, but have impact in ways they’ve never had before.”
Story by Chris Quirk